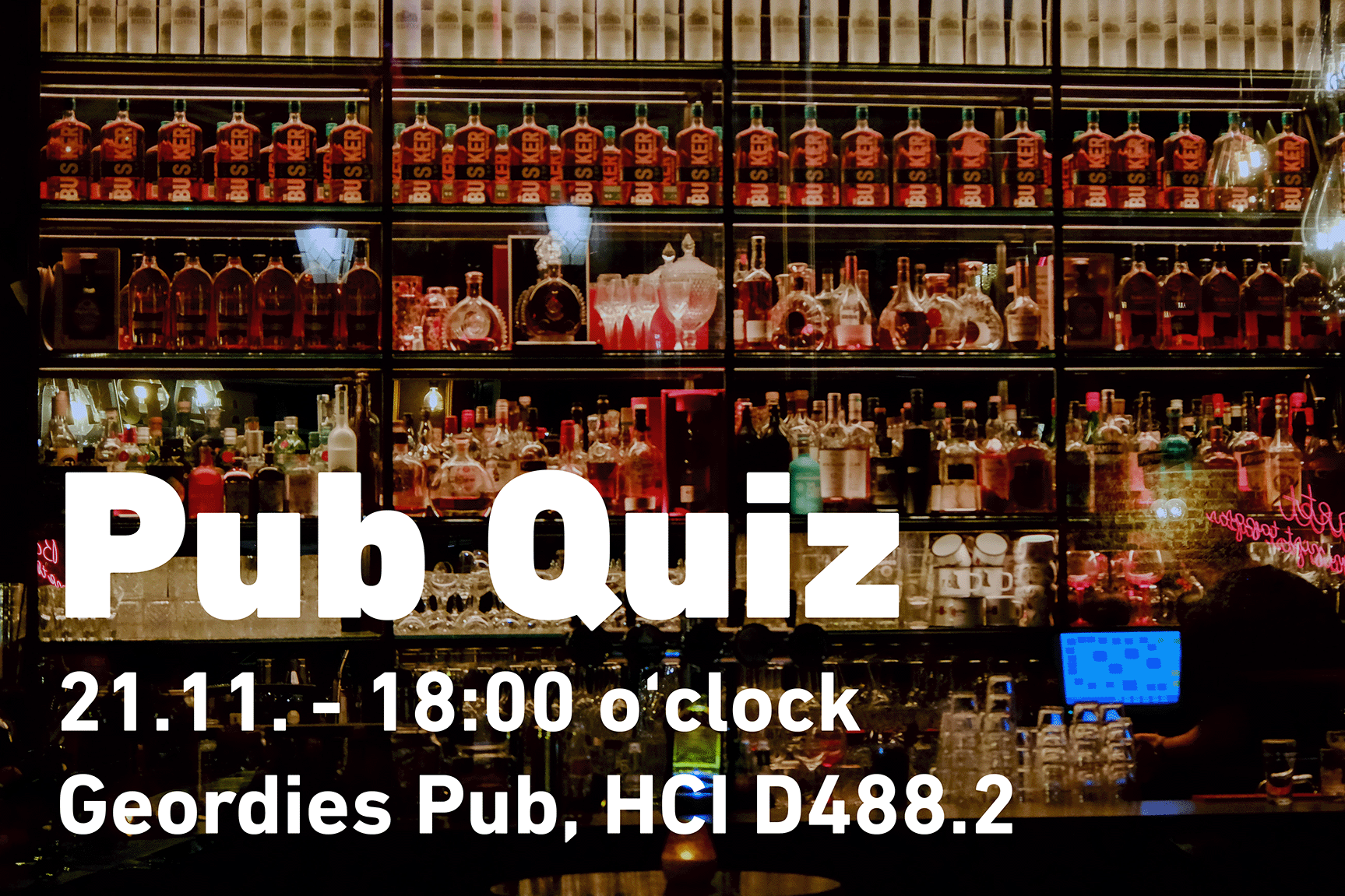
AV-MAVT and SAM Pub Quiz
AV-MAVT and SAM are excited to invite you to a Pub Quiz in the Geordies Pub (HCI D488.2) on the 21.11.24! Test your trivia knowledge and enjoy a fun evening with fellow materials and mechanical engineering students. Event Details: Don’t miss out on this opportunity to show off your trivia knowledge, make new connections, and […]
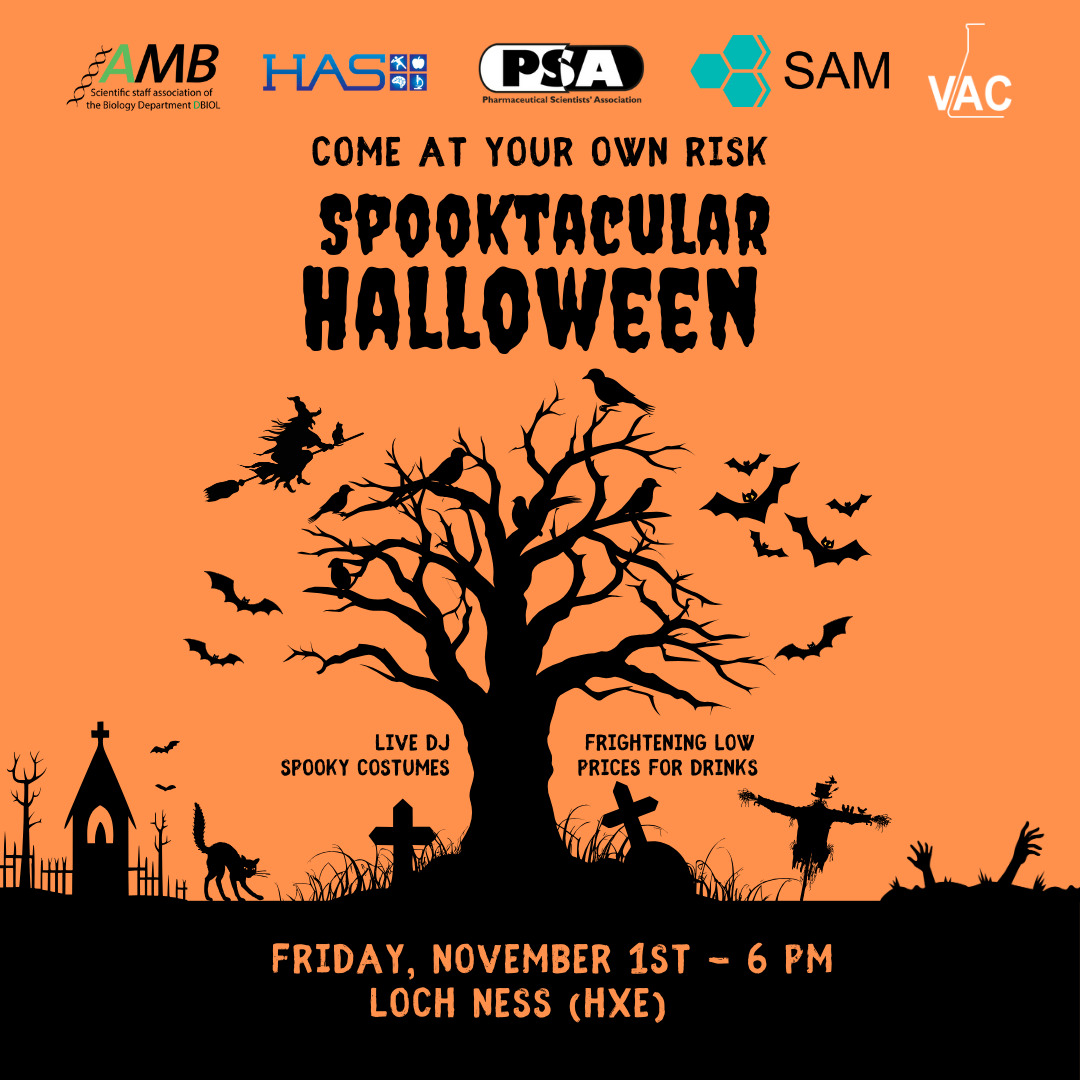
Halloween Party
Join us for a drink or two at our joint Halloween Party with the other Staff Associations of the HCI. Friday, the 1st of November starting at 6 pm at Lochness in HXE.
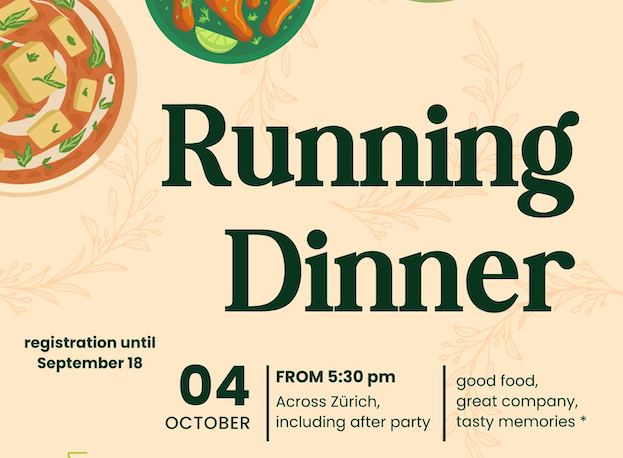
SAM Running Dinner
We are organising a running dinner on the 4th of October, together with our friends from AMB, PSA and VAC. For the ones that don’t know what a running dinner is, don’t worry, there is no running involved. You pair up with a partner and will host one out of three courses (starters, main, dessert) […]
PhD and Postdoc Retreat 2021
More information will follow…
SAM Poker Night 2021
More information will follow…
Feuerzangenbowle 2022
More information will follow…
SAM Pub Quiz Night – Topic: Diversity, Equality, Inclusion 2022
More information will follow…